Thank you for visiting nature.com. You are using a browser version with limited support for CSS. To obtain the best experience, we recommend you use a more up to date browser (or turn off compatibility mode in Internet Explorer). In the meantime, to ensure continued support, we are displaying the site without styles and JavaScript.
Nature Communications volume 13, Article number: 4735 (2022 ) Cite this article Regenerated Cellulose

Molecular conformations induced by the rotation about single bonds play a crucial role in chemical transformations. Revealing the relationship between the conformations of chiral catalysts and the enantiodiscrimination is a formidable challenge due to the great difficulty in isolating the conformers. Herein, we report a chiral catalytic system composed of an achiral catalytically active unit and an axially chiral 1,1′-bi-2-naphthol (BINOL) unit which are connected via a C–O single bond. The two conformers of the catalyst induced by the rotation about the C–O bond, are determined via single-crystal X-ray diffraction and found to respectively lead to the formation of highly important axially chiral 1,1′-binaphthyl-2,2′-diamine (BINAM) and 2-amino-2′-hydroxy-1,1′-binaphthyl (NOBIN) derivatives in high yields (up to 98%), with excellent enantioselectivities (up to 98:2 e.r.) and opposite absolute configurations. The results highlight the importance of conformational dynamics of chiral catalysts in asymmetric catalysis.
Wei Wen, Ming-Jing Luo, … Qi-Xiang Guo
Taku Miyazawa, Takuro Suzuki, … Shigeki Matsunaga
Ze-Shui Liu, Yu Hua, … Qianghui Zhou
Conformations are spatial arrangements of the atoms formed by rotations about a single bond. In most cases, pure conformers cannot be isolated, because the molecules are constantly rotating through all the possible conformations (Fig. 1a). When the rotation about a single bond is restricted, a special class of conformers called atropisomers can be isolated as different chemical species (Fig. 1b). Conformations and rotations about single bonds are crucial to molecular functions and chemical transformations. For example, conformational dynamics play a key role in enzyme catalysis1,2. However, in asymmetric catalysis, it is well-known that structural rigidity of non-enzymatic chiral catalysts, such as the 2,2′-bis(diphenylphosphino)−1,1′-binaphthyl (BINAP)-metal complexes3,4 and BINOL-based phosphoric acids5,6 shown in Fig. 1c, is usually essential in achieving high levels of asymmetric inductions7,8,9 and less attention has been paid to the molecular flexibility and conformational dynamics of the chiral catalysts10,11,12,13,14,15. Due to the great difficulty in isolating the conformers, it is very challenging to reveal the relationship between the conformations of chiral catalysts and the enantiodiscrimination.
a C–C bond rotation in ethane. b Restricted rotation about the C–C single bonds in atropisomers. c Rotation about the C–C single bond locked in BINAP-metal complexes and BINOL-based phosphoric acids. d Design of a chiral catalytic system for conformational enantiodiscrimination enabling asymmetric construction of atropisomers with opposite absolute configurations. BINOL, 1,1′-bi-2-naphthol; BINAM, 1,1′-binaphthyl-2,2′-diamine; NOBIN, 2-amino-2′-hydroxy-1,1′-binaphthyl; BINAP, 2,2′-bis(diphenylphosphino)-1,1′-binaphthyl; Ph, phenyl; CPA, chiral phosphoric acids.
Axially chiral molecules not only are abundant in nature16, but also make great success in many scientific fields such as materials science and asymmetric synthesis especially17,18,19,20,21,22,23,24. BINOL, BINAM, and NOBIN (Fig. 1b) are among the most prominent and valuable axially chiral molecules17,18,19,20,21,22,23,24. Enantiopure (R)- and (S)-BINOL and some of their derivatives are commercially available nowadays, in contrast, enantiopure BINAMs and NOBINs with diverse substitution patterns are still very difficult to obtain despite the fact that great efforts have been devoted to their synthesis in the past three decades22,23,24,25,26,27,28,29,30,31,32,33,34,35,36,37,38,39,40,41,42,43.
In this work, we design a chiral catalytic system (Fig. 1d) which is composed of an achiral catalytically active unit (copper complex of 1,10-phenanthroline unit) and an axially chiral BINOL unit. The two units are connected via a C–O single bond, the rotation about which induces two distinct conformers. This dynamic catalytic system exhibits high activity and excellent enantioselectivity in the atroposelective synthesis of axially chiral BINAM and NOBIN derivatives which are highly important biaryl atropisomers. Moreover, the two conformers of the catalyst are determined via single-crystal X-ray diffraction and the relationship between the favored conformers and the enantiodiscrimination as well as the observed absolute configurations of the two classes of products is revealed.
To commence our investigation, we employed (R)-BINOL as the chiral unit and classical N,N-bidentate 1,10-phenanthroline as the achiral chelating unit. The two units were merged into a novel class of ligands via the formation of a C–O bond between the C2 carbon of the phenanthroline unit and the oxygen of one phenolic hydroxy group (see Section 2.1 in the Supplementary Information (SI) for details). Thus, the ligands are endowed with axial chirality, excellent coordination ability as well as conformational flexibility. In addition, the C9 position of the phenanthroline unit was left to be modified with sterically demanding groups which serve as a shield to narrow the chiral space around the metal center (Fig. 2a). The asymmetric cross-coupling of azonaphthalene 1a with N-benzyl-2-naphthylamine 2a which produces BINAM derivative 3a was selected as the reaction37 to evaluate the ligands (Fig. 2a) and copper, which has never been reported to catalyze this reaction, was employed as the metal.
a Shield screening (48 h). b Influence of the hydroxy group (48 h). c Substrate scope (60 h). Reaction conditions: 1 (0.10 mmol), 2 (0.12 mmol), 1,2-dichlorobenzene (2.0 mL), under air, 30 °C unless noted otherwise (3n: 40 °C). All yields are isolated. Enantiomeric ratios (e.r.) were determined via HPLC analysis and reported as (R:S). Me, methyl; nPr, n-propyl; Bn, benzyl; Ph, phenyl. The absolute configuration of all the products with blue thick bonds is R.
We initiated our study by screening the substituents on the C9 position of the phenanthroline unit to find an appropriate shield (Fig. 2a). Although the reaction could hardly proceed under the optimized conditions (see Section 2.2 in the SI for optimization) when L1 was employed as ligand, the desired product 3a was obtained in 40% yield when L2 which possesses a chlorine atom on the C9 position of the phenanthroline was used as ligand. However, the enantioselectivity is very low (52:48 e.r.). Introducing a phenyl substituent on the C9 position as the shield (L3) can dramatically improve the enantioselectivity (72:28 e.r.). Replacing the phenyl substituent with more sterically hindered 3,5-dimethylphenyl group (L4) did not improve the enantioselectivity. Although replacing it with 3,5-di(trifluoromethyl)phenyl group (L5) improved the yield to 69%, the enantioselectivity is low. After evaluating the ligands (L6-L10) with fused aromatic ring on the C9 position of the phenanthroline, L8 (9-anthracenyl as shield) was found to be the optimal ligand in terms of enantioselectivity, giving the desired product with 97.5:2.5 e.r. and in 67% yield. It is worthy to note that the absolute configuration of the major product is (R). Ligand L11 with the same structure as L8 except that the hydroxy group was converted to the methoxy group, was also tested under the same conditions (Fig. 2b). Surprisingly, both the yield (25%) and the enantioselectivity (47:53 e.r) decreased dramatically. These results indicate that possibly in the transition state hydrogen bond is formed between the substrate and the phenolic hydroxy group.
Further study revealed that extending the reaction time to 60 h increases the yield to 91% and the excellent e.r. (97.5:2.5) remains (Fig. 2c, product 3a) utilizing L8 as ligand. With the best ligand and optimized conditions in hand, we explored the scope of the produced BINAM derivatives (Fig. 2c). Replacing the benzyl moiety in the ester part of azonaphthalene with phenyl group renders 3b in better yield but lower enantioselectivity. Whereas azonaphthalene with n-propyl ester group gives 3c in lower yield with almost same level of enantioinduction (97:3). Azonaphthalenes with bromo, methyl, or ester group on C6 position and with bromo, methyl, phenyl, or methoxy group on C7 position are well tolerated. The corresponding BINAM derivatives 3d–3j were obtained in yields ranging from 72 to 98% and with almost same level of enantioselectivities (from 96.5:3.5 to 97.5:2.5 e.r.). Introducing substituents on C6 or C7 position of 2-naphthylamines exhibits neglectable effect on the e.r. (ranging from 96:4 to 98:2) and products 3k-3q were successfully obtained in moderate to excellent yields. 2-Naphthylamines with other similar protecting groups were also examined and all the corresponding products 3r-3v were produced in excellent enantioselectivities (from 96.5:3.5 to 97.5:2.5 e.r.) and moderate to excellent yields. Furthermore, the catalyst is also compatible with various substituents in both azonaphthalenes and 2-naphthylamines simultaneously. Highly enantioenriched (from 96:4 to 98:2 e.r.) disubstituted BINAM derivatives 3w-3ad have been successfully synthesized in good to excellent yields. To study the practicability of the protocol, a reaction was carried out on one-gram scale using 1a (1.00 g, 3.45 mmol) and 2a as reactants (Section 2.4 in the SI), producing 3a in 79% yield and with excellent e.r. (97:3). In addition, product 3a was successfully transformed to (R)-BINAM in 85% yield with the same e.r. (97:3) through Raney nickel-catalyzed hydrogenation under 1 atm (Section 2.5 in the SI). Thus, this catalytic system proves to be efficient for asymmetric synthesis of BINAM derivatives.
Encouraged by the previous results, we then targeted the asymmetric cross-coupling of azonaphthalene 1a with 2-naphthol 4a which produces NOBIN derivative 5a (Fig. 3a)37. When the optimal ligand L8 in the previous cross-coupling of azonaphthalenes with 2-naphthylamines was employed, in combination with Cu(acac)2 to in-situ prepare the catalyst, good yield (70%) but poor enantioselectivity (66:34 e.r.) was obtained. The absolute configuration of the major product is determined to be (R), which is the same as those of products 3 in cross-coupling of azonaphthalene 1a with N-benzyl-2-naphthylamine 2a (Fig. 2). Since 2-naphthol is an acidic substrate, the hydrogen bonding may be interrupted which may partly account for the observed low enantioinduction. Both the yield (78%) and the enantioselectivity (68.5:31.5) are slightly improved in the presence of 20 mol% NaHCO3, possibly due to an enhanced hydrogen bonding (see Supplementary Table 8). Interestingly, ligand L11 gives better results (89% yield and 24.5:75.5 e.r.) compared with L8. More importantly, the absolute configuration of the major product is (S). We speculate that in the cross-coupling of azonaphthalenes with 2-naphthols, the azonaphthalene is activated by the copper center and could react with 2-naphthol directly. Hydrogen bonding interaction is not indispensable in the transition state, and the steric repulsion generated from the methoxy group may shift the conformational equilibrium of the ligand and favor the distribution of conformation-B. Therefore, to enhance the dominance of conformation-B so as to obtain more efficient enantiodiscrimination, more sterically hindered tert-butyldimethylsilyl, triisopropylsilyl, and tert-butyldiphenylsilyl groups were employed to protect the phenolic hydroxy group, and ligands L12–L14 were synthesized and evaluated (Fig. 3a). All these three ligands display excellent yields and enantioselectivities (e.r. up to 2.5:97.5 for L13). These results are consistent with the designed conformation-controlled enantiodiscrimination model (Fig. 1d) and the observed absolute configuration of the major product.
a Influence of the conformational equilibrium on enantiodiscrimination. b Substrate scope. Reaction conditions: 1 (0.10 mmol), 4 (0.12 mmol), Cu(acac)2 (10 mol%), ligand (12 mol%), m-xylene (2.0 mL), under N2, at 25 °C. All yields are isolated. Enantiomeric ratios (e.r.) were determined via HPLC analysis and reported as (R:S). Me, methyl; nPr, n-propyl; iPr, iso-propyl; Bn, benzyl; Ph, phenyl. The absolute configuration of all the products with red thick bonds is S.
This catalytic system (L13/Cu(acac)2) was further evaluated with various substituted azonaphthalenes and 2-naphthols (Fig. 3b). In most of the cases, the transformations proceeded smoothly and the corresponding NOBIN derivatives were obtained in excellent yields and enantioselectivities under the optimized conditions (see Section 3.2 in the SI for optimization). Replacing the benzyl moiety in the ester part of azonaphthalene with n-propyl or iso-propyl group provides the products 5b and 5c respectively, in slightly reduced yields and enantioselectivities. Azonaphthalenes with bromo, methyl or phenyl at either C7 or C6 position of the naphthalene ring are compatible, affording the corresponding products (5d-5i) in good to excellent yields (83–97%) and excellent e.r. (up to 3.5:96.5). Introducing a methyl ester group on the C6 position gave the product (5j) in 97% yield and with an e.r. of 3:97. In addition, the effect of substituents on the 2-naphthol were also examined. 2-Naphthols with bromo, methyl, phenyl, cyano or methoxy group on either C7 or C6 position were tolerated well, and products 5k-5t were produced in good to excellent yields (84–98%) and enantioselectivities (up to 3.5:96.5 e.r.). Methyl ester, formyl and cyclohexyl groups on C6 position of 2-naphthol are also compatible, affording product 5u in 98%, 5v in 75%, and 5w in 87% yield respectively with almost identical level of enantioinductions. More importantly, substituents (bromo, methyl, especially phenyl) on C3 position of 2-naphthol were well-tolerated, rendering the products (5x-5z) in good to excellent yields and enantioselectivities. Substrates with methyl, bromo, or methyl ester group on both azonaphthalenes and 2-naphthol were tested and corresponding NOBIN derivatives (5aa-5ad) were successfully synthesized with good to excellent enantioinductions. A gram-scale reaction for asymmetric cross-coupling of 1a (1.16 g, 4.00 mmol) and 4a was also carried out (Section 3.4 in the SI), producing 5a in 96% yield and with excellent e.r. (6:94). Furthermore, product 5a can be transformed to (S)-NOBIN in 96% yield and with 6:94 e.r. through Raney nickel-catalyzed hydrogenation (Section 3.5 in the SI). Therefore, the catalytic system can also be applied to the atroposelective construction of NOBIN derivatives.
To determine the major conformations of this chiral catalytic system induced by the rotation about the C–O bond, single-crystal X-ray diffraction was employed to determine the structure of copper complexes prepared from the optimal ligands. A single crystal (Cu-1) was successfully obtained by using 2-(anthracen-9-yl)−9-chloro-1,10-phenanthroline (L15) to stabilize the catalyst prepared from L8 and Cu(MeCN)4PF6. As shown in Fig. 4a, two distinct conformers of L8 induced by the rotation of the carbon-oxygen single bond which connects the BINOL unit with the phenanthroline unit, were observed in the crystal structures (corresponding to two copper complexes co-crystallized in 1:1 ratio). In Cu-1(A) the hydroxy group points towards the front (conformation-A) and the anthracene group of the stabilizer L15 is under the phenanthroline unit. In Cu-1(B), the hydroxy group extends towards the back (conformation-B) and the anthracene group of L15 is above the phenanthroline unit. This indicates the conformations of the ligand could discriminate the coordination modes of the substrate with the catalyst if we imagine that substrate 1a would take the place of L15 during the catalytic process. Since previous results proved the hydroxy group is crucial to the reactivity and enantioselectivity, conformation-A shown in Cu-1(A) is believed to be favorable and productive. By reacting L13 with CuCl (1.0 equiv.) in methanol and recrystallizing from dichloromethane/n-hexane, a copper complex composed of Cu(L13)2 and CuCl2 was obtained. The crystal structure of Cu-2 in Fig. 4b shows two L13 coordinates to one copper in a criss-cross pattern, forming a C2-symmetric complex in which the bulky triisopropylsilyl groups point away from the metal center (similar to the conformation-B shown in Cu-1(B)). Conformation-A was not observed in the solid state of L13, and conformation-B is proposed to be the favored one during catalysis. A shift in the conformational equilibrium of the ligands may occur when the steric hindrance of R1 increases (Figs. 1d and 3a). These crystallographic data are in agreement with the proposed possible transition states (TS-A and TS-B) shown in Fig. 1d and the observed conformation-controlled enantiodiscrimination in the two reactions for the asymmetric construction of BINAM and NOBIN derivatives (Figs. 2 and 3).
a Copper complex Cu-1 prepared from L8, Cu(MeCN)4PF6 and 2-(anthracen-9-yl)−9-chloro-1,10-phenanthroline (L15). b Copper complex Cu-2 prepared from L13 and CuCl.
In summary, conformational flexibility has been incorporated into the design and development of a chiral catalytic system which proves to be efficient and highly enantioselective for the atroposelective synthesis of highly valuable axially chiral BINAM and NOBIN derivatives. The relationship between the conformational preference of the catalysts and the conformation-controlled enantiodiscrimination has been revealed. The absolute configuration of the products is determined by the conformation of the catalysts rather than the absolute configuration of the BINOL unit. The findings in this study highlight the importance of conformational dynamics of chiral catalysts in asymmetric catalysis and may inspire future development of other chiral catalysts.
To a solution of Cu(MeCN)4PF6 (3.7 mg, 0.010 mmol, 10 mol%) and L8 (7.7 mg, 0.012 mmol, 12 mol%) in 1,2-dichlorobenzene (2.0 mL) were added azo compound 1 (0.10 mmol) and the 2-naphthylamine derivative 2 (0.12 mmol). The mixture was stirred under air at 30 °C for 60 h. Upon completion, the resulting mixture was directly purified by flash chromatography on silica gel using petroleum ether/ethyl acetate as the eluent to afford the desired products 3.
To a solution of Cu(acac)2 (2.6 mg, 0.010 mmol, 10 mol%) and L13 (9.6 mg, 0.012 mmol, 12 mol%) in m-xylene (2.0 mL) were added azo compound 1 (0.10 mmol) and the 2-naphthylamine derivative 4 (0.12 mmol). The mixture was stirred under N2 atmosphere at 25 °C for 12 h. Upon completion, the resulting mixture was directly purified by flash chromatography on silica gel using petroleum ether/ethyl acetate as the eluent to afford products 5.
The data supporting the findings of this study are available within the paper and its Supplementary Information. Metrical parameters for the structure of copper complexes (Cu-1 and Cu-2 in Fig. 4) (see Supplementary Information) are available free of charge from the Cambridge Crystallographic Data Centre (https://www.ccdc.cam.ac.uk/) under reference numbers CCDC 2096699 and CCDC 2096715, respectively. Any further relevant data are available from the authors on request.
Bhabha, G. et al. A dynamic knockout reveals that conformational fluctuations influence the chemical step of enzyme catalysis. Science 332, 234–238 (2011).
Article ADS CAS PubMed PubMed Central Google Scholar
Hammes, G. G. Multiple conformational changes in enzyme catalysis. Biochemistry 41, 8221–8228 (2002).
Article CAS PubMed Google Scholar
Miyashita, A. et al. Synthesis of 2,2′-bis(diphenylphosphino)-1,1′-binaphthyl (BINAP), an atropisomeric chiral bis(triaryl)phosphine, and its use in the rhodium(I)-catalyzed asymmetric hydrogenation of α-(acylamino)acrylic acids. J. Am. Chem. Soc. 102, 7932–7934 (1980).
Berthod, M., Mignani, G., Woodward, G. & Lemaire, M. Modified BINAP: the how and the why. Chem. Rev. 105, 1801–1836 (2005).
Article CAS PubMed Google Scholar
Akiyama, T., Itoh, J., Yokota, K. & Fuchibe, K. Enantioselective Mannich-type reaction catalyzed by a chiral Brønsted acid. Angew. Chem., Int. Ed. 43, 1566–1568 (2004).
Uraguchi, D. & Terada, M. Chiral Brønsted acid-catalyzed direct Mannich reactions via electrophilic activation. J. Am. Chem. Soc. 126, 5356–5357 (2004).
Article CAS PubMed Google Scholar
List, B. & Yang, J. W. The organic approach to asymmetric catalysis. Science 313, 1584–1586 (2006).
Article CAS PubMed Google Scholar
Yoon, T. P. & Jacobsen, E. N. Privileged chiral catalysts. Science 299, 1691–1693 (2003).
Article ADS CAS PubMed Google Scholar
Zhou, Q.-L. (ed.) Privileged Chiral Ligands and Catalysts (Wiley-VCH, Weinheim, 2011).
Crawford, J. M. & Sigman, M. S. Conformational dynamics in asymmetric catalysis: is catalyst flexibility a design element? Synthesis 51, 1021–1036 (2019).
Article CAS PubMed PubMed Central Google Scholar
Li, H., Liu, X., Wu, F., Tang, L. & Deng, L. Elucidation of the active conformation of cinchona alkaloid catalyst and chemical mechanism of alcoholysis of meso anhydrides. Proc. Natl Acad. Sci. U.S.A. 107, 20625–20629 (2010).
Article ADS CAS PubMed PubMed Central Google Scholar
Knowles, R. R. & Jacobsen, E. N. Attractive noncovalent interactions in asymmetric catalysis: Links between enzymes and small molecule catalysts. Proc. Natl Acad. Sci. U.S.A. 107, 20678–20685 (2010).
Article ADS CAS PubMed PubMed Central Google Scholar
Sohtome, Y., Tanaka, S., Takada, K., Yamaguchi, T. & Nagasawa, K. Solvent-dependent enantiodivergent Mannich-type reaction: utilizing a conformationally flexible guanidine/bisthiourea organocatalyst. Angew. Chem. Int. Ed. 49, 9254–9257 (2010).
Storch, G. & Trapp, O. Temperature-controlled bidirectional enantioselectivity in a dynamic catalyst for asymmetric hydrogenation. Angew. Chem. Int. Ed. 54, 3580–3586 (2015).
Stone, E. A. et al. Isolating conformers to assess dynamics of peptidic catalysts using computationally designed macrocyclic peptides. ACS Catal. 11, 4395–4400 (2021).
Article CAS PubMed PubMed Central Google Scholar
Bringmann, G., Gulder, T., Gulder, T. A. M. & Breuning, M. Atroposelective total synthesis of axially chiral biaryl natural products. Chem. Rev. 111, 563–639 (2011).
Article CAS PubMed Google Scholar
Cheng, J. K., Xiang, S.-H., Li, S., Ye, L. & Tan, B. Recent advances in catalytic asymmetric construction of atropisomers. Chem. Rev. 121, 4805–4902 (2021).
Article CAS PubMed Google Scholar
Wencel-Delord, J., Panossian, A., Leroux, F. R. & Colobert, F. Recent advances and new concepts for the synthesis of axially stereoenriched biaryls. Chem. Soc. Rev. 44, 3418–3430 (2015).
Article CAS PubMed Google Scholar
Lin, P. (ed.) 1,1′-Binaphthyl-Based Chiral Materials: Our Journey (Imperial College Press, London, 2009).
Brunel, J. M. BINOL: a versatile chiral reagent. Chem. Rev. 105, 857–897 (2005).
Article CAS PubMed Google Scholar
Chen , Y. , Yekta , S. & Yudin , AK Modified BINOL ligands in asymmetric catalysis .Chem.Rev. Fr.103, 3155–3212 (2003).
Article CAS PubMed Google Scholar
Kočovský, P., Vyskočil, Š. & Smrčina, M. Non-symmetrically substituted 1,1′-binaphthyls in enantioselective catalysis.Chem.Roar.103, 3213–3245 (2003).
Article PubMed CAS Google Scholar
Ding, K., Li, X., Ji, B., Guo, H. & Kitamura, M. Ten years of research on NOBIN chemistry. Curr. Org. Synth. 2, 499–545 (2005).
Ding, K., Guo, H., Li, X., Yuan, Y. & Wang, Y. Synthesis of NOBIN derivatives for asymmetric catalysis. Top. Catal. 35, 105–116 (2005).
Smrčina, M., Lorenc, M., Hanuš, V. & Kočovský, P. A facile synthesis of 2-amino-2′-hydroxy-1,1′-binaphthyl and 2,2′-diamino-1,1′ -binaphthyl by oxidative coupling using copper(II) chloride.Son.Lett.1991, 231–232 (1991).
Singer, R. A. & Buchwald, S. L. Preparation of 2-amino-2′-hydroxy-1,1′-binaphthyl and N-arylated 2-amino-1,1′-binaphthyl derivatives via palladium-catalyzed amination. Tetrahedron Lett. 40, 1095–1098 (1999).
Yusa, Y., Kaito, I., Akiyama, K. & Mikami, K. Asymmetric catalysis of homo-coupling of 3-substituted naphthylamine and hetero-coupling with 3-substituted naphthol leading to 3,3′-dimethyl-2,2′-diaminobinaphthyl and -2-amino-2′-hydroxybinaphthyl. Chirality 22, 224–228 (2010).
De, C. K., Pesciaioli, F. & List, B. Catalytic asymmetric benzidine rearrangement. Angew. Chem. Int. Ed. 52, 9293–9295 (2013).
Li, G.-Q. et al. Organocatalytic aryl−aryl bond formation: an atroposelective [3,3]‑rearrangement approach to BINAM derivatives. J. Am. Chem. Soc. 135, 7414–7417 (2013).
Article CAS PubMed Google Scholar
Shirakawa, S., Wu, X. & Maruoka, K. Kinetic resolution of axially chiral 2-amino-1,1′-biaryls by phase-transfer-catalyzed N-allylation. Angew. Chem. Int. Ed. 52, 14200–14203 (2013).
Lu, S., Poh, S. B. & Zhao, Y. Kinetic resolution of 1,1′-biaryl-2,2′-diols and amino alcohols through NHC-catalyzed atroposelective acylation. Angew. Chem. Int. Ed. 53, 11041–11045 (2014).
Patel, DC et al.Gram scale conversion of R‐BINAM to R‐NOBIN.J. Org.Chem.81, 1295–1299 (2016).
Article CAS PubMed Google Scholar
Chen , Y.-H. , Qi , L.-W. , Fang , F. & Tan , B. Organocatalytic atroposelective arylation of 2-naphthylamines as a practical approach to axially chiral biaryl amino alcohols .Angew.Chem.Int.Ed.56, 16308–16312 (2017).
Forkosh, H., Vershinin, V., Reiss, H. & Pappo, D. Stereoselective synthesis of optically pure 2‑amino-2′-hydroxy-1,1′-binaphthyls. Org. Lett. 20, 2459–2463 (2018).
Article CAS PubMed Google Scholar
Lu, S., Poh, S. B., Rong, Z.-Q. & Zhao, Y. NHC-catalyzed atroposelective acylation of phenols: access to enantiopure NOBIN analogs by desymmetrization. Org. Lett. 21, 6169–6172 (2019).
Article CAS PubMed Google Scholar
Wang, D., Liu, W., Tang, M., Yu, N. & Yang, X. Atroposelective synthesis of biaryl diamines and amino alcohols via chiral phosphoric acid catalyzed para-aminations of anilines and phenols. iScience 22, 195–205 (2019).
Article ADS CAS PubMed PubMed Central Google Scholar
Qi, L.-W., Li, S., Xiang, S.-H., Wang, J. & Tan, B. Asymmetric construction of atropisomeric biaryls via a redox neutral cross-coupling strategy. Nat. Catal. 2, 314–323 (2019).
Lu, S. et al. Practical access to axially chiral sulfonamides and biaryl amino phenols via organocatalytic atroposelective N-alkylation. Nat. Commun. 10, 3061 (2019).
Article ADS PubMed PubMed Central CAS Google Scholar
Yang, G., Guo, D., Meng, D. & Wang, J. NHC-catalyzed atropoenantioselective synthesis of axially chiral biaryl amino alcohols via a cooperative strategy. Nat. Commun. 10, 3062 (2019).
Article ADS PubMed PubMed Central CAS Google Scholar
Liu, W., Jiang, Q. & Yang, X. A versatile method for kinetic resolution of protecting-group-free BINAMs and NOBINs through chiral phosphoric acid catalyzed triazane formation. Angew. Chem. Int. Ed. 59, 23598–23602 (2020).
Ding, W.-Y. et al. DFT-guided phosphoric-acid-catalyzed atroposelective arene functionalization of nitrosonaphthalene. Chem 6, 2046–2059 (2020).
Zhao, X.-J. et al. Enantioselective synthesis of 3,3′-disubstituted 2-amino-2′-hydroxy-1,1′-binaphthyls by copper-catalyzed aerobic oxidative cross-coupling. Angew. Chem. Int. Ed. 60, 7061–7065 (2021).
Dyadyuk, A. et al. A chiral iron disulfonate catalyst for the enantioselective synthesis of 2-amino-2′-hydroxy-1,1′-binaphthyls (NOBINs). J. Am. Chem. Soc. 144, 3676–3684 (2022).
Article CAS PubMed Google Scholar
We thank the Research Center of Analysis and Test, East China University of Science and Technology for the help on the characterization, and Prof. Yifeng Chen (ECUST) for sharing the instruments. We thank Prof. Yuan-Yuan Zhu (HFUT) and Prof. Jie Sun (SIOC) for the help with single crystal X-ray diffraction and structure analysis. We acknowledge the National Natural Science Foundation of China (21702059), Fundamental Research Funds for the Central Universities (222201814014, JKVJ1211010, JKVJ12001010), Shanghai Pujiang Program (18PJ1402200), Shanghai Municipal Science and Technology Major Project (2018SHZDZX03), Program of Introducing Talents of Discipline to Universities (B16017) and the “Thousand Plan” Youth program for financial support.
These authors contributed equally: Shouyi Cen, Nini Huang.
Key Laboratory for Advanced Materials and Joint International Research Laboratory of Precision Chemistry and Molecular Engineering, Feringa Nobel Prize Scientist Joint Research Center, Frontiers Science Center for Materiobiology and Dynamic Chemistry, School of Chemistry and Molecular Engineering, East China University of Science & Technology, Shanghai, 200237, China
Shouyi Cen, Nini Huang, Dongsheng Lian, Ahui Shen, Mei-Xin Zhao & Zhipeng Zhang
You can also search for this author in PubMed Google Scholar
You can also search for this author in PubMed Google Scholar
You can also search for this author in PubMed Google Scholar
You can also search for this author in PubMed Google Scholar
You can also search for this author in PubMed Google Scholar
You can also search for this author in PubMed Google Scholar
Z.Z. conceived of and directed the project. S.C. developed the BINAM part, N.H. developed the NOBIN part, S.C. and D.L. performed the gram-scale reactions. S.C., N.H., A.S., M.X.Z. and Z.Z. cowrote the manuscript.
Correspondence to Mei-Xin Zhao or Zhipeng Zhang.
The authors declare no competing interests.
Nature Communications thanks Jung Woon Yang, and the other, anonymous, reviewer(s) for their contribution to the peer review of this work. Peer reviewer reports are available.
Publisher’s note Springer Nature remains neutral with regard to jurisdictional claims in published maps and institutional affiliations.
Open Access This article is licensed under a Creative Commons Attribution 4.0 International License, which permits use, sharing, adaptation, distribution and reproduction in any medium or format, as long as you give appropriate credit to the original author(s) and the source, provide a link to the Creative Commons license, and indicate if changes were made. The images or other third party material in this article are included in the article’s Creative Commons license, unless indicated otherwise in a credit line to the material. If material is not included in the article’s Creative Commons license and your intended use is not permitted by statutory regulation or exceeds the permitted use, you will need to obtain permission directly from the copyright holder. To view a copy of this license, visit http://creativecommons.org/licenses/by/4.0/.
Cen, S., Huang, N., Lian, D. et al. Conformational enantiodiscrimination for asymmetric construction of atropisomers. Nat Commun 13, 4735 (2022). https://doi.org/10.1038/s41467-022-32432-8
DOI: https://doi.org/10.1038/s41467-022-32432-8
Anyone you share the following link with will be able to read this content:
Sorry, a shareable link is not currently available for this article.
Provided by the Springer Nature SharedIt content-sharing initiative
By submitting a comment you agree to abide by our Terms and Community Guidelines. If you find something abusive or that does not comply with our terms or guidelines please flag it as inappropriate.
Nature Communications (Nat Commun) ISSN 2041-1723 (online)
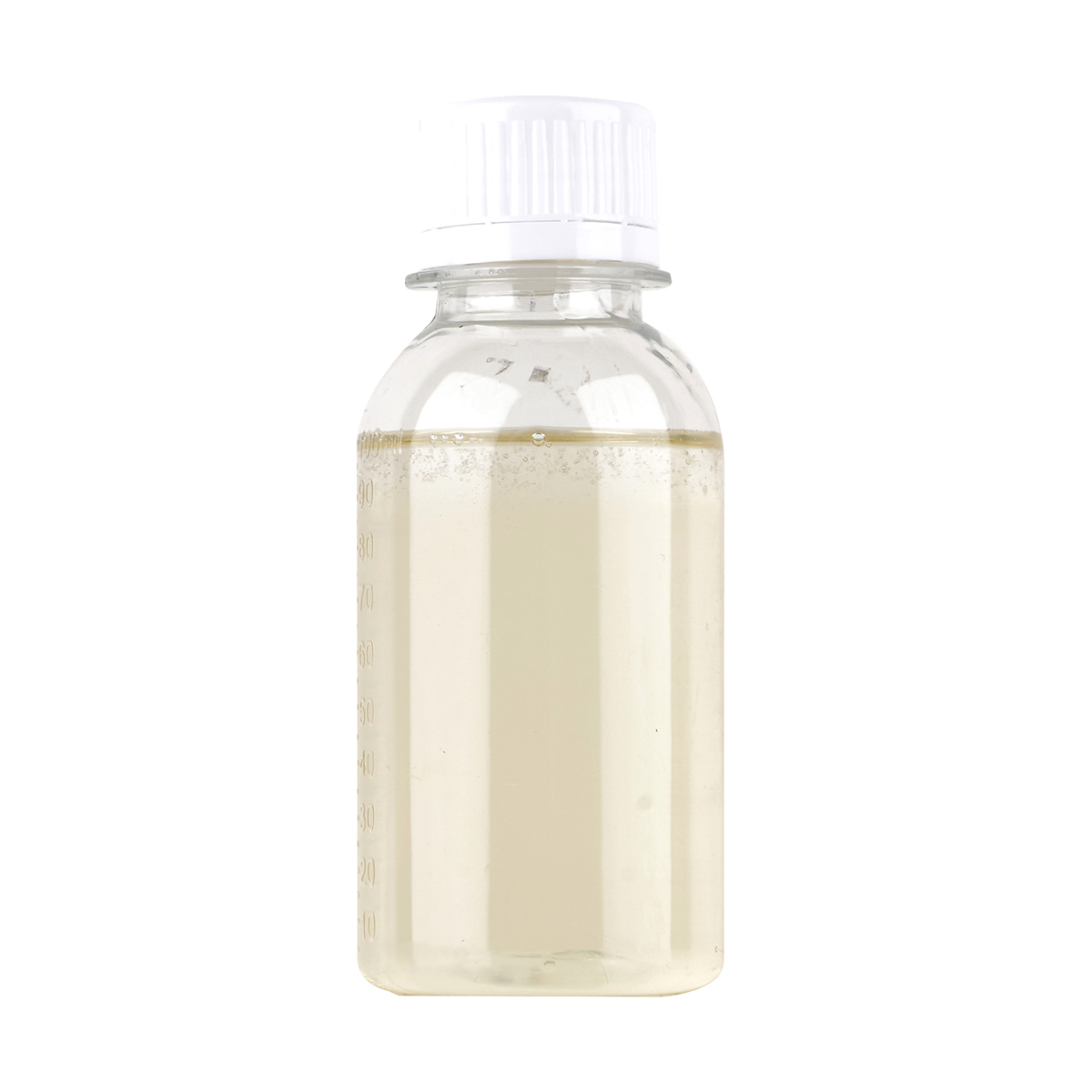
H 1/4 Nitrocellulose Sign up for the Nature Briefing newsletter — what matters in science, free to your inbox daily.